Mission Statement
Currently, the large majority of control laws employed by the European Aeronautics, Space and Defense industries is still designed and analyzed using predominantly linear control theory. However, these linear controllers often do not provide sufficient robustness and performance when approaching the physical limits of the aircraft, where nonlinear effects dominate and strong coupling has to be considered. This is for example the case, when flying complex, highly curved three dimensional trajectories and when damages or faults occur.
In order to allow flying under these conditions, the controller has to be able to fully utilize the available control authority and redundancy while simultaneously adhering to the current flight envelope limits. Nonlinear control theory provides the necessary methods to analyze and design such controllers.

The philosophy adopted by the Nonlinear Adaptive Flight Control (NAFC) group splits the overall control task into two different assignments. At first, a baseline controller is designed such that desired robustness and performance criteria are achieved under nominal flight conditions. However, aircraft operate in highly uncertain environments, are subject to modeling uncertainties, sensor imperfections and unknown exogenous disturbances. In the second step the baseline controller is augmented by an adaptive controller, which shall reduce and/or cancel the impact of uncertainties.
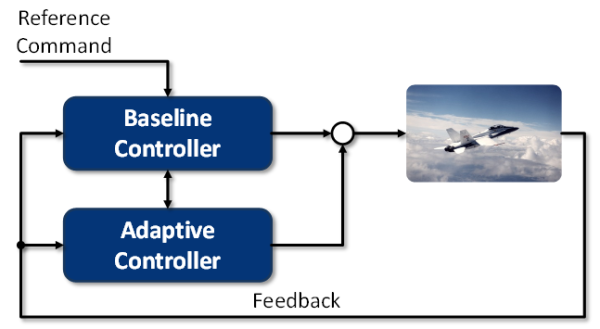
Linear and Nonlinear Baseline Controller
Since each flying platform has its own inherent dynamics and must comply with precise requirements, the baseline controller must be tailored for a particular system and developed in accordance with specific mission requirements. The control designer has currently multiple baseline strategies at his disposal. These can be split into linear (e.g. Linear Quadratic Regulator or Linear Quadratic Gaussian) and nonlinear baseline control techniques (e.g. feedback linearization-based control laws or backstepping).
In order to fully exploit the physical capabilities of an aerial system, the NAFC group focuses its efforts in researching and designing nonlinear baseline control laws. This derives from the fact that flying platforms are systems, which present complex and highly nonlinear dynamics, i.e. this kind of baseline controller is considered more suitable for the task at hands.
One of the main research topics currently pursued by the NAFC group when dealing with feedback linearization and backstepping-based baseline controllers is precisely the design and implementation of nonlinear reference models. The objective of a reference model is to shape the commanded control variables (e.g. guidance, stick inputs) in a physically feasible way. Unlike their nonlinear counterparts, linear reference models approximate the plant characteristics only in regions where the plant dynamics are considered linear. In the case of aerial applications, such regions are obviously quite small. This means that the use of nonlinear reference models might substantially improve the closed-loop performance.
Applied Methods: Nonlinear Dynamic Inversion, Backstepping, Command-Filtered Backstepping.
Augmentation
In case of off-nominal, adverse flight conditions, for example due to malfunctions or damages, the baseline controller is often not able to ensure safe flight anymore. In this situation, an adaptive augmentation of the baseline controller may help to either recover the desired performance or, in the latter case, to provide an adequate level of performance in order to maintain safe flight. These capabilities have been demonstrated in numerous flight tests and thus highlight that adaptive control is indeed beneficial for the overall system performance as well as the overall system safety. Nevertheless, the transition of adaptive control into an accepted and widely used technology for the control of safety-critical systems such as aircraft is still an on-going process. Current research at the Institute of Flight Systems Dynamics in the field of adaptive control aims at contributing to this transition.
A fundamental challenge in adaptive control is its diversity. For the augmentation of a baseline controller, numerous adaptive control architectures and modifications exist, each of which with distinct advantages and disadvantages. In order to systematically choose the appropriate adaptive controller based on the mission requirements at hand, one research focus of the Nonlinear Adaptive Flight Control group is the systematic assessment of adaptive control architectures. For this assessment, one also requires methods to analyze their robustness and the performance. The development of appropriate, non-conservative methods constitutes another research area. Ultimately, these methods will also be an integral part of future certification strategies of adaptive control.
While adaptive control may compensate for large uncertainties in theory, in practice, its capabilities are naturally limited by the aircraft physics and the actuation system. Innovative approaches of adhering to these envelope limits are another area of ongoing research. In this context, fault tolerant control to compensate for degradations of the actuation system is an especially challenging task as it simultaneously requires the compensation of the degradation as well as a dynamic adjustment of the envelope limits.
Applied Methods: Model Reference Adaptive Control (MRAC), Adaptive Control, Retrospective Cost Adaptive Control, Neural Networks, Concurrent Learning, Pseudo Control Hedging, Gaussian Processes MRAC
Illustrational Video: Example for uncertainty compensation – Neural Network approximation of a nonlinear function
Simulation and Flight Testing
The choice of employed baseline and adaptive control methods is mainly driven by the type of application. Therefore, real flight tests are particularly important and are conducted with a variety of aerial vehicles, i.e. fixed-wing airplanes, multirotors and missiles.
» Learn more
The video below shows flight tests with a path control for multirotor UAVs, based on nonlinear dynamic inversion and pseudo control hedging.
Prior to real test flights, each control algorithm is extensively tested in a high-fidelity simulation environment that covers all effects which are present in the real system.

Team
You want to learn more about what we are doing or you are interested in cooperating with us? Contact any one of us:
Michael KrenmayrSelected Publications
Path Generation and Control for Unmanned Multirotor Vehicles Using Nonlinear Dynamic Inversion and Pseudo Control Hedging", in 19th IFAC Symposium on Automatic Control, 2013. | , "|
Optimizing Reference Commands for Concurrent Learning Adaptive Optimal Control of Uncertain Dynamical Systems", in AIAA Guidance, Navigation, and Control (GNC) Conference, 2013. | , "|
Nonlinear Position Control Approaches for Quadcopters using a Novel State Representation", in AIAA Guidance, Navigation and Control Conference, 2012. | , "
Header Image: A. Heddergott / TUM